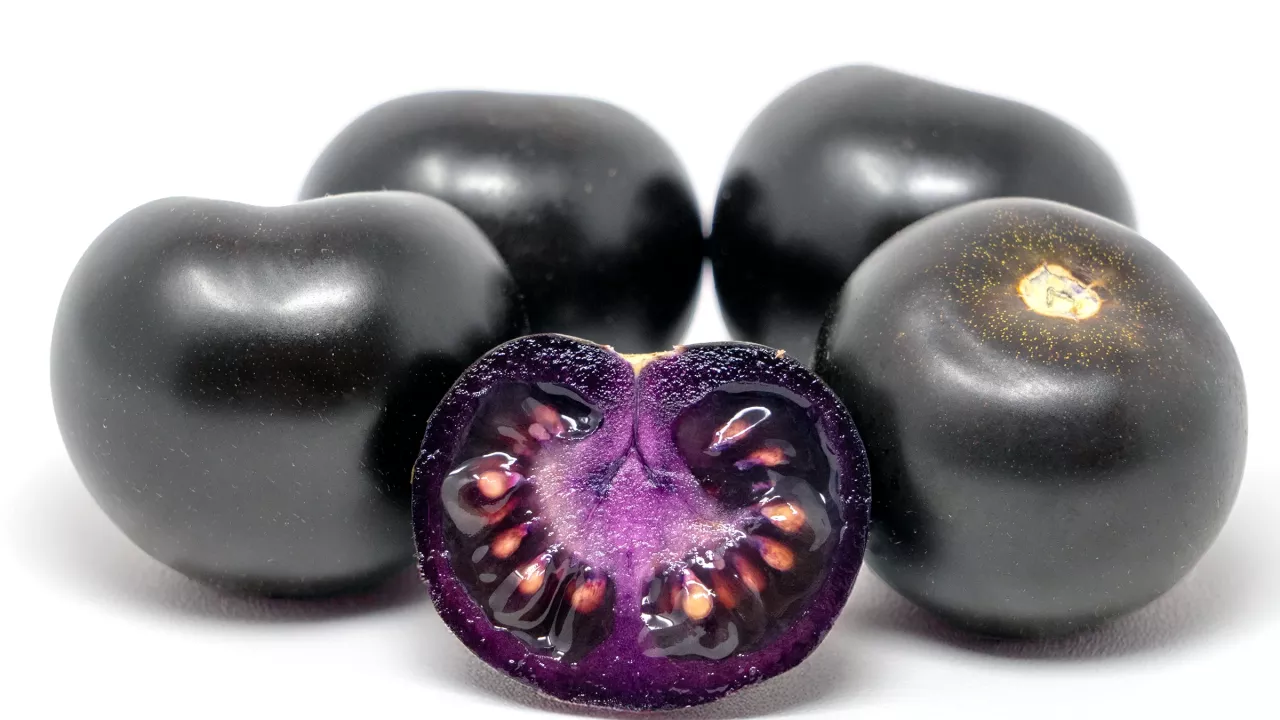
How Biotechnology is Reshaping Our Plates
Introduction
The world faces unprecedented challenges to food security and sustainability. Rapid climate change is altering growing seasons, increasing the frequency of extreme weather events, making crops more sensitive to disease, and shifting growing regions—all of which can directly reduce the amount of food we are able to grow and harvest. At the same time, the global population is projected to reach nearly 10 billion by 2050 (The United Nations, Department of Economic and Social Affairs), and the demand for food grows disproportionately as societies become increasingly industrialized (Fukase and Martin, 2024). Decreased supply and increased demand mean we need to expand global food production by 60-70% by 2050 to avoid widespread food insecurity (FAO, 2017).
However, agriculture is already one of the largest contributors to environmental degradation. Industrial farming practices drive deforestation, emit significant amounts of greenhouse gases, deplete freshwater resources, and erode soil health. For example, agriculture accounts for approximately 25% of global greenhouse gas emissions and 70% of freshwater withdrawals (IPCC, 2019; FAO, 2020). This creates a feedback loop: as the global population grows and demands more food, agriculture intensifies, further degrading the environment, and further limiting our ability to grow food. To sustainably meet future food demands, we must not only increase yields but also radically reduce the environmental impact of agricultural systems by cutting carbon emissions, conserving land, and improving resource use efficiency. What can drive such radical change? In the view of a growing number of researchers, the answer might be genetic engineering.
What is genetic engineering?
Genetic engineering is a process used to change an organism’s DNA to introduce new traits or enhance existing ones. There are two main ways this can be done. First, scientists can transfer beneficial genes from one organism into another—essentially copying and pasting useful adaptations into crops that we already rely on. This approach results in what are called transgenic crops, where a gene from a different species is added. Second, genetic engineering can be used to mimic or speed up the process of natural evolution by introducing targeted or random mutations and then screening for desirable traits, such as increased resilience or productivity. This approach includes techniques like CRISPR-Cas9 (which yields gene-edited crops) or chemically induced random mutagenesis, and does not necessarily involve foreign DNA, making it more similar to traditional breeding but with greater precision and speed.
With sufficient mastery, this can be extremely powerful for improving crops. Our planet is incredibly diverse, with plants from hot, dry deserts to icy, cold tundras having already solved the challenges posed by these extreme conditions. If we can transfer these resilient, wild plant traits into our domesticated crops, we can help protect our food from the effects of climate change.
Alternatively, genetic engineering could be used to enhance the nutritional profile of crops. Most countries face some vitamin or mineral deficiency, regardless of wealth. This has been exacerbated by breeding crops to maximize the mass of each harvest, decreasing the concentration of nutrients in our food over time (Sharff et al, 2021). Using genetic engineering, we could rev up the pathways for generating micronutrients without reducing yield.
However, genetically modified organisms (GMOs) evoke controversy, and the conversation around them is nuanced. While the technology itself offers significant benefits, valid concerns regarding its implementation persist. These include the monopolization of elite crop lines by large corporations, limiting access for smaller farmers, and the environmental consequences of some GMO-associated agricultural practices, such as indiscriminate pesticide and herbicide spraying. Despite these challenges, the ethical and inclusive development of GMOs could unlock their potential to address global food challenges.
A brief history of genetic engineering in crops
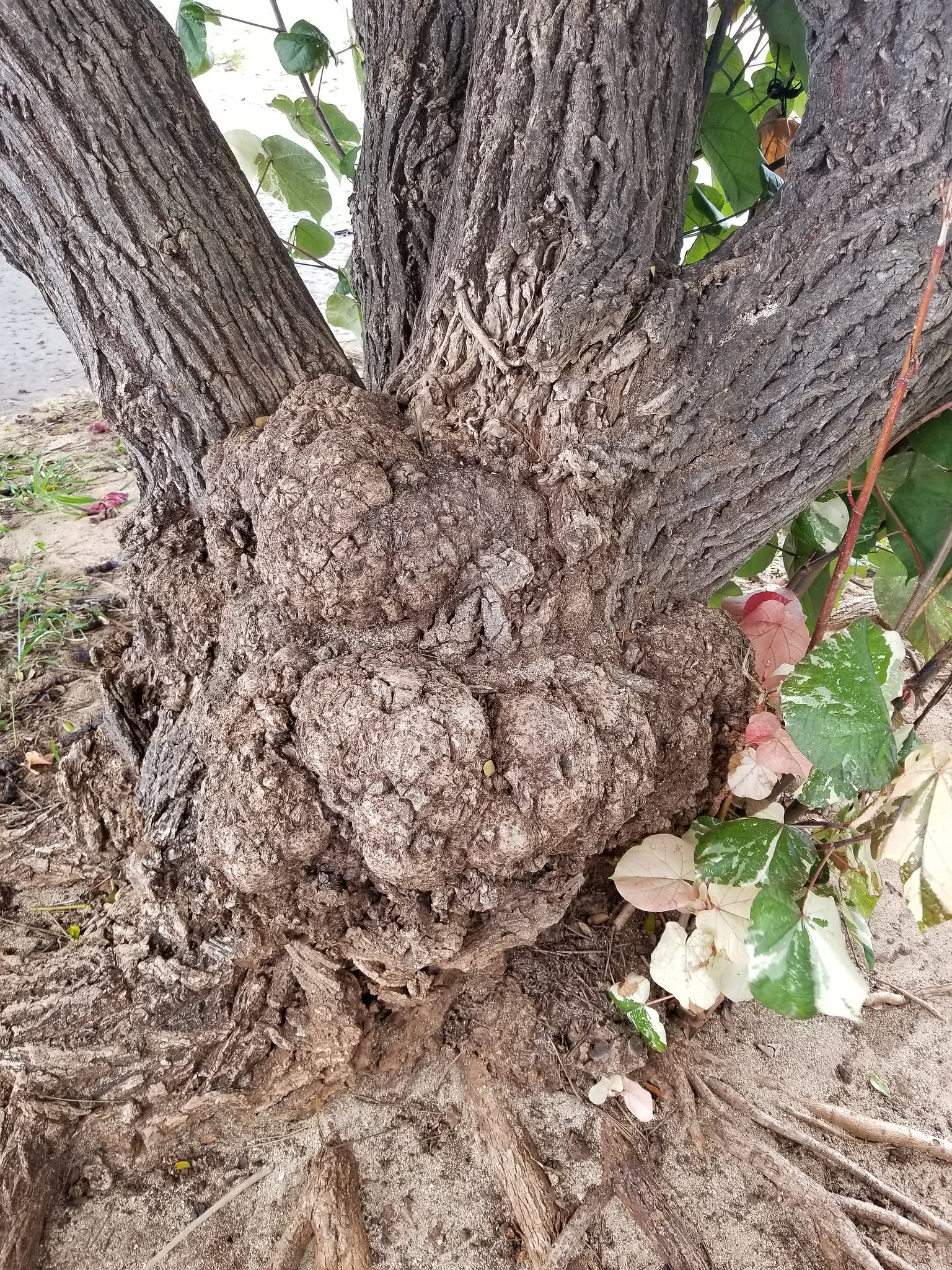
Genetic engineering in agriculture began with the groundbreaking work of Marc Van Montagu and Jeff Schell in the early 1970s. They discovered that Agrobacterium tumefaciens, a naturally occurring plant pathogen, could transfer part of its DNA (known as the T-DNA) into a plant’s genome, causing crown gall disease. This finding laid the foundation for using Agrobacterium as a tool for genetic modification. Building on this discovery, Mary-Dell Chilton, along with her team at Washington University in the late 1970s, sought to determine if the DNA that Agrobacterium transfers could be replaced with a different genetic sequence. Chilton’s landmark experiment demonstrated this concept by successfully introducing foreign DNA into a plant genome, proving that Agrobacterium could be engineered to carry and deliver beneficial traits instead of pathogenic ones (Chilton et al., 1977). This breakthrough marked the first step toward the genetic modification of plants, transforming the scientific and agricultural landscape.
The first genetically modified crop to hit the market was the Flavr Savr tomato, introduced in the 1990s, which was designed to resist rotting and extend its shelf life. This marked the beginning of commercial GM crop adoption. Soon after, Bt cotton entered the market—engineered to produce proteins from Bacillus thuringiensis that are toxic to specific pests, reducing the need for chemical insecticides. Roundup Ready crops followed, allowing farmers to control weeds more efficiently using herbicides. These early GMOs were widely adopted in large-scale agriculture and became profitable staples in global farming.
At the same time, genetic engineering was also being applied to solve urgent agricultural and nutritional problems. In the 1990s, the Hawaiian papaya industry was on the verge of collapse due to the Papaya Ringspot Virus, but a GM papaya engineered for viral resistance was approved and freely distributed, saving the crop. Later, Golden Rice was developed by biofortifying rice with provitamin A to address severe vitamin A deficiency in developing countries—a deficiency responsible for hundreds of thousands of child deaths and cases of blindness annually (Black et al., 2008). These innovations highlight the humanitarian potential of GM technology beyond commercial gains.
More recently, CRISPR-Cas9 technology has revolutionized genetic engineering by enabling precise edits to plant genomes. Unlike traditional GMOs that often introduce foreign genes in random genome locations, CRISPR allows scientists to "edit" a plant’s DNA or add small, targeted modifications. While technically more limited than introducing whole genes, it mimics the natural genome mutation and selection process undertaken during traditional breeding, just with much more precision. Commonly, these edits are so-called "knockout" mutations, where CRISPR is used to mutate a gene, making it dysfunctional.
You might be asking: how could knocking out a gene improve a crop? One example is knocking out genes that pathogens use to gain access to plant cells (aka, susceptibility genes), which could enhance crop resistance to pests and disease, thereby reducing the need for insecticides or fungicides. Another common example of CRISPR-edited crops is non-browning potatoes and apples, in which enzymes responsible for oxidizing sugars are knocked out, reducing food waste. On a broader scale, there are several genes crops contain that equipped them for successful reproduction in the wild, genes that are simply unnecessary or incompatible with cultivation for human needs. Targeted elimination of these genes can increase yields while improving the sustainability of crops.
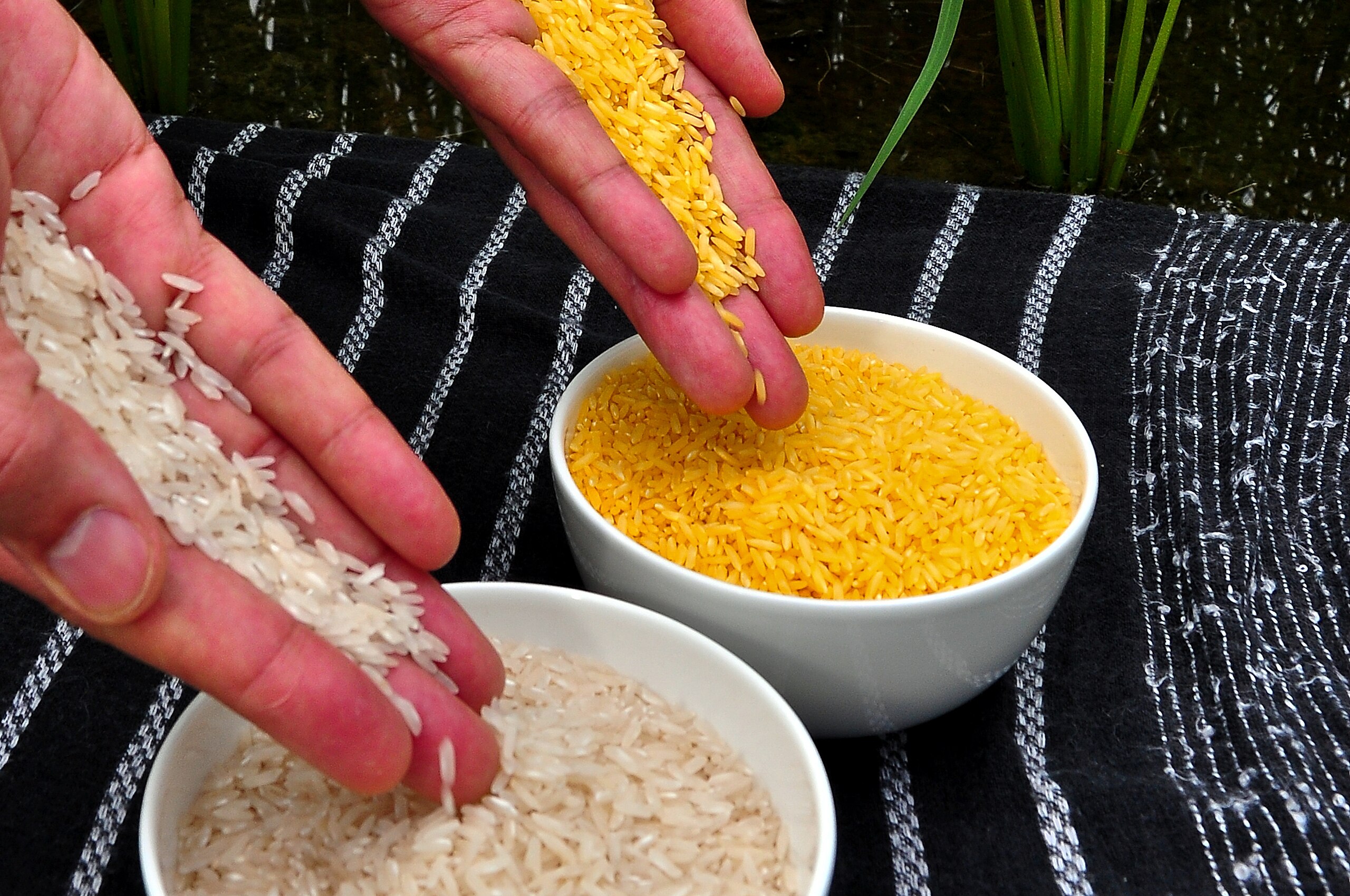
Recent developments in GM crops
The popularity of GM crops has been quite volatile. After an initial boom and then bust, they are now on a more sustainable trajectory of adoption. With the market now looking more promising, more and more biotech startups are introducing products to smaller consumer markets. As representatives, let's take a look at two colorful examples: The Purple Tomato and the Pinkglow Pineapple.
The Purple Tomato is a deep purple variety of tomatoes engineered to overproduce a natural purple pigment in plants called anthocyanins. These are the same pigments that give blackberries and eggplant their color, and they are potent antioxidants that have been associated with reduced disease in humans. Tomatoes already produce anthocyanins (you can see these in the stems of seedlings, which are often purple), but the production of these pigments is typically turned down in the fruits. 20 years ago, leading scientist Cathie Martin and her team transferred a gene that turns on anthocyanin production from snapdragon flowers to tomatoes (Butelli et al., 2008). The result is striking, with these purple tomatoes containing as much anthocyanin as blueberries or eggplants by weight.
The Purple Tomato is the first GM crop marketed directly to at-home gardeners. Norfolk Healthy Produce, the Davis-based company distributing the Purple Tomato in the US, sells seed packets directly to consumers. These can be treated as heirloom varieties since the tomatoes will grow true to seed if you save them. If you just want to try the fruit, you can pick some up at the Davis Farmer's Market, as well as at select stores.
Pinkglow Pineapple is another example of a charismatic GM fruit sold directly to customers. Sold by Del Monte, these pineapples have been engineered to produce more lycopene, the antioxidant pigment that makes tomatoes red, by reducing the activity of an enzyme that converts lycopene into beta-carotene. While you can't legally grow this one yourself, the bright pink color of the pineapple makes for a novel experience as a treat.
What the future holds
Genetic engineering is an incredible feat that holds much promise for the future. The first wave of GM crops picked the low-hanging, money-making fruit by engineering cash crops to resist pests and herbicides, creating something close to a monopoly on major crops like maize, soybean, and cotton. The next wave will be different. Dozens, if not hundreds of companies are popping up with innovative and diverse ideas to engineer crops with greater yields and less input. As some examples: Hundreds of scientists are currently working on developing climate change resilient crops, others are working on transferring the nitrogen-fixing traits of legumes or bacteria to crops to reduce the use of greenhouse gas-intensive fertilizer, and photosynthesis itself is being engineered to increase efficiency to allow crops to fix more carbon on the same land. Taking it further, crops will be engineered to supply a greater amount of nutrients, making a balanced diet more accessible across the globe. Other plants may even be used for bioremediation, cleaning up heavy-metal contamination, or acting as biosensors for other contaminants.
The new wave of GM crops under development is better conceptualized under the term GMOrganics, crops engineered to outgrow weeds, resist disease and extreme weather, self-fertilize, improve soil biodiversity, and be more nutritious, all without synthetic inputs. By leveraging genetic engineering, we can remodel our agricultural system to be healthier and more sustainable.
Comments